By Hugh Ross,
Ice ages are rare in Earth’s history; ice age cycles are even rarer. In the past 2 billion years, ice ages occurred in only 3 of the 21 geological periods prior to our own (Quaternary), and those ice ages were relatively brief, with each one briefer than the last. The Quaternary is the only other geological period of the past 2 billion years during which ice sheets persisted. For the past 2.59 million years, thick ice sheets have covered at least 10 percent (and as much as 23 percent) of the continents.
One extraordinary feature of the present glacial age is that it occurs during life’s history when the Sun is at its brightest (see figure 1).1 Furthermore, it occurs when the atmospheric greenhouse gas abundance is 280 parts per million, no less than it was during the Neogene period (beginning 23 million years ago and ending 3 million years ago).
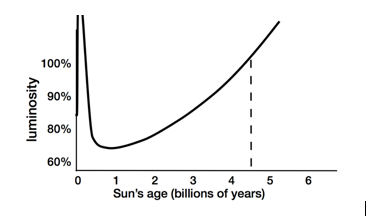
The present glacial age is also cyclical. Ice coverage of the continents oscillates rhythmically from 10 to 23 percent within either a 41,000- or 100,000-year period. Only during the last 0.00057 part of its history has Earth manifested an ice age cycle. Only during the last 0.00017 part of Earth’s history has the ice age cycle encompassed a 100,000-year period. As this article will explain, a 100,000-year ice age cycle is essential to permitting the existence of a large human population capable of launching and sustaining civilization.
Perfect Storm
The current delicate balance between ice and no ice, manifesting itself as an ice age cycle, is due to a “perfect storm” of five amazing and simultaneous tectonic events. First, the breakup of the supercontinent Pangaea resulted in a remarkable alignment of continents. Landmasses, not oceans, came to dominate the high latitudes of the northern hemisphere (see figure 2) and the Arctic Ocean became a nearly enclosed sea. Because continents have a much lower heat capacity compared to oceans, such a continental arrangement dramatically cooled the high northern latitudes and played an important role in the formation of the polar ice cap.

Second, Antarctica split off from Australia and centered on the South Pole, completely surrounded by open ocean (see figure 3).2 Between 2.75 and 3.15 million years ago, about 8 million cubic kilometers (2 million cubic miles) of additional ice was deposited on Antarctica.3 This increased ice coverage and Antarctica’s geographical location initiated stronger heat transfer from the North Atlantic Ocean to the deep parts of the Pacific Ocean.4 This heat transfer substantially cooled the northern hemisphere and, thus, contributed to its glaciation.
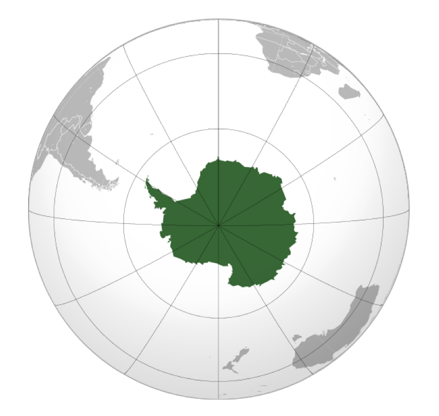
Third, about 3 million years ago, converging tectonic plates led to the formation of a land bridge connecting North and South America. This isthmus cut off water flow between the Pacific and Atlantic Oceans, which forced a rerouting of ocean currents and atmospheric circulation. These new currents and circulations provided a more even distribution of precipitation on the continents, a crucial requirement for the launch and spread of future civilization.
Forcing currents and wind patterns more into north-south, rather than east-west, directions resulted in global cooling. Specifically, the Gulf Stream brought atmospheric moisture into the Arctic region, contributing to the formation of ice caps over the Arctic Ocean and Greenland.
Fourth, the movement and uplift of Greenland also contributed to the establishment of the current ice age cycle. Recently, four geophysicists demonstrated that over the past 60 million years Greenland has moved northward by 18° latitude (see figure 4).5 They showed that a “northward rotation of the entire mantle and crust toward the pole, dubbed True Polar Wander,” moved Greenland 12° north.6 Plate tectonic reconstruction moved Greenland relative to the mantle another 6° north. All this movement resulted in Greenland becoming the northernmost landmass on Earth. Its northern tip is just 713 kilometers (443 miles) from the North Pole.

While Greenland’s new location certainly made it much colder, the movement alone is insufficient to explain its enormous store of ice. The four geophysicists also discovered that, as Greenland moved north, a mantle plume pulse thinned the lithosphere (crust) below it. Starting about 5 million years ago, younger plume pulses led to dramatic uplift. These pulses lifted the eastern parts of Greenland to elevations exceeding 3,000 meters (10,000 feet) above sea level.
The combination of Greenland’s northerly location and high elevation above sea level decreased its temperatures enough to sustain long-term glaciation. Today, an ice sheet covers 81 percent of Greenland and has reflected more of the Sun’s heat and light, thereby cooling the entire planet.
Fifth, the continuing collision of the Indian subcontinent with Eurasia (see figure 5) resulted in the uplift of the Tibetan Plateau. About 50 million years ago, the Indian tectonic plate hit the Eurasian tectonic plate at a hyper-fast velocity of 18–20 centimeters per year (7–8 inches per year).7 Then, about 23 million years ago, the sea gap between India and Asia closed and the Himalayas began to rise up. The northeast movement of the Indian subcontinent into Eurasia has now slowed to about 5 centimeters per year.
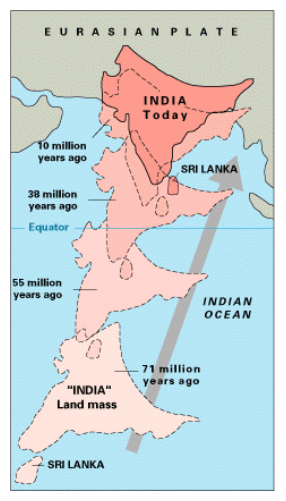
Around 8 million years ago, the ongoing collision between India and Asia lifted the Tibetan Plateau to an average elevation above 2,300 meters (7,500 feet). This large, high plateau created an air pressure gradient relative to the nearby oceans and initiated the Indian and East Asian monsoons.8 As the uplift continued, the monsoons strengthened. At 2.59 million years ago, the ongoing collision lifted the Tibetan Plateau to an average elevation of about 4,000 meters (13,125 feet).9 This average height was sufficient enough to form permanent ice, alter the summer-winter monsoon cycle,10 and cause loess (wind-blown fine dust) sediments to be deposited all over the lowlands of China.11
Today, even though Earth is in a warm interglacial, the store of ice in the Himalayas and the Tibetan Plateau is greater than 14,000 cubic kilometers,12 exceeded only by the ice caps over Antarctica and Greenland. The Tibetan Plateau presently stands at an average elevation of 4,600 meters (15,100 feet).
When covered in snow and ice, the Tibetan Plateau, because of its low latitude (26–37° above the equator), has a powerful cooling impact on the planet. It reflects solar heat four times as efficiently as an equivalent area of ice-covered arctic landmass. Many climatologists are convinced that the rise of the Tibetan Plateau was the predominant factor in triggering the onset of the current ice age.13 Indeed, Tibet may have been the first region in the world to experience large-scale galaciation.14
Given how bright the Sun is today, it is truly remarkable that Earth is presently experiencing an ice age. Nothing less than the just-right combination and near-simultaneous occurrence of the five factors described above would be adequate to produce an ice age at this time. However, the present ice age is much less extensive than ones occurring previous to 3 million years ago. and the minimal nature of the present ice age is what makes its cyclical property possible.
How to Generate a Cyclical Ice Age
Milankovitch cycles, the collective effect of periodic changes in Earth’s orbit and rotation on its climate, drive the cyclical property of the current ice age. The cyclical change in the direction of Earth’s rotation axis relative to the stars is called precession. Currently, Earth’s axis points toward Polaris. In about 13,000 years it will point toward Vega, and in another 13,000 years it will again point to Polaris.
There is also a precession effect related to Earth’s orbit around the Sun. The net outcome is that within a cyclical period of 21,600 years, Earth’s northern hemisphere alternates between pointing toward and away from the Sun during the northern hemisphere winter. For example, in 2014, Earth was most distant from the Sun on July 3, which explains why high northern latitude landmasses (e.g., England) experience milder seasonal differences than do high southern latitude landmasses (e.g., southern Chile).
Earth’s rotation axis tilt (obliquity) relative to the plane of its orbit varies between 22.1° and 24.5° within a period of 41,000 years. The greater the tilt, the greater the temperature difference between summers and winters worldwide. Given the current sizes and configurations of the continents, a lesser obliquity generates a global cooling effect whereas a greater obliquity has a global warming effect.
Like all planets, Earth’s orbit has an elliptical shape. Earth’s eccentricity (departure of the orbital ellipse from circularity) varies between 0.000055 and 0.0679 due to the gravitational influence of the neighboring gas giant planets. Earth’s two most dominant eccentricity cycles last about 400,000 years and 100,000 years, respectively.
Typically, these cycles would have a subtle influence on global mean temperatures. However, when the planet is as delicately balanced as it is now, between an ice age and no ice age, the impact is much bigger.
Several events worked together to tip the balance from an ice-free planet to one with extensive ice:
- The movement of a large continent to the South Pole and several other continents to high northern latitudes
- Greenland’s movement toward the North Pole and tectonic uplift
- The near enclosure of the Arctic Ocean
- The joining together of North and South America
- The rise of the Tibetan Plateau
The result is permanent ice formed over a portion of Antarctica and intermittent small patches of ice over high-latitude and high-elevation parts of the northern continents in response to the Milankovitch cycles.
The ice age cycle that occurred around 0.86–0.95 to 2.59 million years ago lasted 41,000 years.15 During that time, the Himalayas and the Tibetan Plateau continued their rise, eventually taking the ice coverage of that region from just 2–4 percent at glacial maximum to about 29–40 percent.16 Consequent increase in solar heat reflection lowered temperatures and resulted in other northern hemisphere snow-and-ice patches growing larger with each successive cycle.
At 0.86–0.95 million years ago, the ice age cycles switched from 41,000 years to 100,000 years. Climatologists have found two contributing causes for this switch. One research team, in their analysis of data from South Atlantic deep-sea cores, discovered that a major shift in Atlantic Ocean thermohaline (heat + salt) circulation occurred about 900,000 years ago.17 The team then demonstrated that this change would have facilitated the drawdown of atmospheric carbon dioxide that occurred at the same time, which in turn would have helped to cool Earth sufficiently to cause the ice age cycle switch.
Other researchers pointed to geological evidence showing that while the Tibetan Plateau steadily rose from a 4,000- to a 4,600-meter average elevation over the past 2.59 million years, there were brief episodes of especially dramatic uplift.18 This quickened uprising explains the intensification of Indian and East Asian monsoonal events19 and light eolian dust accumulation20 that occurred about 0.9 million years ago.
High-resolution magnetostratigraphy and tectonosedimentology shows such uplift took place about 0.8 million years ago.21 This event caused snow and ice to cover the majority of the plateau at glacial maxima.22 The coverage, combined with heightened atmospheric dust, brought on enough global cooling to significantly enlarge the snow-and-ice packs at high northern latitudes. Photosynthetic activity decreased and intensified monsoons enhanced silicate weathering, both contributing to a drawdown in atmospheric carbon dioxide.
The intensified cooling stabilized the 100,000-year ice age cycle. Figure 6 shows Antarctic temperature changes for the last four ice age cycles. In response to the ever-increasing elevation of the Himalayas and the Tibetan Plateau, each successive ice age over the past 800,000 years became slightly more severe.

About 100,000 years ago, the Tibetan Plateau experienced a tectonic uplift event of the same order of magnitude as the one it experienced about 0.8–0.9 million years ago.23 Consequently, the most recent ice age was the most expansive of all (see figure 7). The larger ice sheets ranged from 1 to 3 kilometers thick.24 Ice covered the entire Antarctic continent and much of the surrounding seawater, and as much as 2.4 million square kilometers of the Tibetan Plateau was under a thick ice sheet. The North American ice sheet reached as far south as 38° north latitude (the Ohio River) while the European ice sheet reached down to 48° north latitude (southern Ukraine). The Tasmanian and Patagonian ice sheets reached up to 41° south latitude.
Additionally, several major ice sheets covered the mountains and surrounding regions of the Andes in South America, the Sierra Nevada and Sierra Madre in North America, the Alps and the Caucasus in Europe, Kilimanjaro and the Atlas in Africa, the Japanese Alps, the Altai and the Tien Shan in Central Asia, the Zagros in Western Asia, Taiwan, and the mountainous regions of New Guinea.

Benefits of Cyclical Ice Ages
During the last ice age cycle, the global ice volume reached maximal about 21,000 years ago. By about 12,000 years ago, most of this ice (excepting that over Antarctica, Greenland, and the Arctic) had melted and global mean temperatures had stabilized. From 120,000–12,000 years ago, Earth’s climate was so variable as to render the launch of human civilization impossible (see figures 8 and 9).25 As one research team demonstrated, the “last glacial climates were extremely hostile to agriculture.”26 The climate variability ending at the same time the warm interglacial began made possible a rapid launch of and increased sophistication in agriculture and civilization. Thus, humanity was poised to grow.
Fields of ice from the last ice age still continue to melt, feeding the rivers that water the great agricultural plains upon which 7 billion people and their animals depend for their food. For example, many rivers in Asia—including the Ganges, Indus, and Yangtze—flow out from the ice fields of the Himalayas and the Tibetan Plateau. These rivers irrigate agricultural plains that sustain nearly 3 billion people. Likewise, glacier melt in several other mountain ranges throughout the rest of the world sustain many populations.
From the high plateaus and slopes exposed at the close of the last ice age, wind-blown dust (loess) provides many of the crucial nutrients that make the lowland plains fertile. The retreating of the ice fields and glaciers concentrated many mineral ores and exposed many other valuable mineral deposits. Thus, the ending of the last ice age contributed greatly to launching and sustaining metallurgy.
As the vast ice sheets covering much of the northern hemisphere’s continents retreated, they scoured out numerous basins. These basins created millions of large lakes. Canada alone possesses about 32,000 such lakes larger than 3 square kilometers. Freshwater produced by the last ice age covers about 9 percent of Canada’s total surface area.
All this surface freshwater area results in evaporation and condensation that produces precipitation over large swaths of land that otherwise would be barren deserts. The lakes and rivers form habitats for marsh plants (such as wild rice) and animals, including fish, crustaceans, waterfowl, and various mammals. The lakes and rivers provided humans with efficient transportation corridors that made possible specialized agriculture and industry. Water flowing through lakes and rivers also made possible the generation of vast networks of hydroelectric power, without which aluminum likely would never have ever been mass produced and sold at an affordable price. The last ice age also carved out a large number of safe, deep-water harbors all over the world.
Thanks to the last ice age, humans were able to migrate from their locale of origination and easily colonize six of the seven current continents. The last ice caused sea levels to fall to about 125 meters (410 feet) below current levels, which resulted in land bridges joining Siberia to Alaska, Britain to France, Asia Minor to the Greek Peninsula, Korea to Japan, Denmark to Sweden, and Vancouver Island and the Queen Charlottes to the British Columbia mainland. It also yielded easy island hops between South East Asia and Australia. These needed migration routes allowed humans to settle in all the temperate and tropical continents prior to the launch of civilization.
Of course, colonization also required that the continents be at such a distance from one another that the ice age cycle could close and open the land bridges and that mountain elevation would tend to rise the closer one got to the equator. By this means melting ice and snow could be more evenly distributed over the continental landmasses. Also, as noted already, it was crucial that the sizes, shapes, and positions of the continents at the time of human habitation be exactly what and where they are today.
There is also an aesthetic benefit from the last ice age. Earth today is blessed with the most spectacular and beautiful scenery it has ever possessed. The receding ice sheets and glaciers have enhanced the relief of Earth’s mountains and valleys. They have created stunning waterfalls. They have nurtured an enormous number of meadows, forests, rivers, and lakes filled with an extravagant variety of plants and animals that display every imaginable combination of color and texture. The ice age cycle’s simultaneous occurrence with such unique tectonic events as the collision of India with Eurasia, the formation of “rings of fire” (chains of volcanoes), the formation of abundant island volcanoes, and the positioning of the continental landmasses also enhanced Earth’s beauty.
I wouldn’t be surprised if all this stunning natural beauty helps people maintain their sanity in today’s high technology and high stress society. To put it another way, it seems that God not only optimally designed Earth and all life to provide for our physical needs, but also designed it to provide for our aesthetic needs. Table 1 summarizes the known benefits of the ice age cycle.
Table 1: Ice Age Cycle Benefits for Humanity
- Melting ice fields brought nutrient-rich alluvial silt to the plains.
- Wind-blown dust delivered other nutrients to the plains.
- Melting glaciers water the plains.
- Ice field and glacier retreat formed millions of lakes.
- Formations of lakes and connecting rivers transformed barren deserts into productive land regions.
- Geological relief yielded abundant hydropower resources.
- Retreating ice sheets formed land bridges warm enough to facilitate human migration.
- Glacial retreat formed many safe harbors.
- Retreating ice sheets, ice fields, and glaciers formed rich ore deposits.
- Retreating ice sheets, ice fields, and glaciers made possible enhanced abundance of plants and animals during the warm interglacial episodes.
- Retreating ice sheets, ice fields, and glaciers created spectacular scenery.
The Long Cool Summer
Figure 6 shows the variation of Earth’s temperature throughout the past four ice age cycles. This graph reveals a cycle of long glacial epochs (80,000–90,000 years) followed by a brief warm interglacial era (10,000–20,000 years). The interglacial is characterized by a rapid temperature rise to a level about 3–4° Centigrade above the current level followed immediately by an equally rapid temperature drop that brings on another long glacial eon. This pattern is seen throughout the entire Quaternary era (the past 2.59 million years), throughout all the ice age cycles, with just one exception.
The one exception is the warm interglacial we currently are experiencing. For our interglacial the temperature indeed rose rapidly following the last glacial maximum (see figure 8). From 9,000 to 12,500 years ago, the global mean temperature rose 8° Centigrade (14.5° Fahrenheit). However, rather than continuing to spike up another 3–4° Centigrade, Earth’s temperature plateaued. All the ice cores from central Antarctica and north central Greenland (see figure 9) show that, for the past 9,000 years, the planet has experienced a steady global mean temperature that happens to be maximally optimal for human civilization. Climatologists refer to the past 9,000 years as the long cool summer, the long warm spring, or simply as the long summer.


Scientists have yet to determine the causes of the long cool summer with any degree of confidence. However, there is no doubt that this climate’s nature is exceptional. In the context of everything else that is essential to support human civilization, it is a unique event in Earth’s history that cannot be sustained much longer and is unlikely to reoccur.
Why Are We Here Now?
The remarkable circumstances in which we presently find ourselves raises the question of why we are here with such a bountiful array of resources at such an amazingly optimal moment in life’s long history. The Bible provides answers.
A big clue comes from noting that God’s work of redemption preceded His work of creation. As Paul declares in his letters to Timothy and Titus:
He has saved us and called us to a holy life—not because of anything we have done but because of his own purpose and grace. This grace was given us in Christ Jesus before the beginning of time. (2 Timothy 1:9)
Paul, a servant of God and an apostle of Jesus Christ to further the faith of God’s elect and their knowledge of the truth that leads to godliness—in the hope of eternal life, which God, who does not lie, promised before the beginning of time. (Titus 1:1–2)
All of God’s works of creation are in the context of His greater works of redemption, the recipients of which include “a great multitude that no one could count, from every nation, tribe, people and language, standing before the throne and in front of the Lamb” (Revelation 7:9). The numbering system of the first-century Greeks extended to the hundreds of millions. Thus, it could be that the “great multitude that no one could count” implies that at least several billion humans will be redeemed. This number in turn implies that Earth’s carrying capacity today would need to support billions of people.
The answer to the question of why we’re here now is to encourage as many individuals as are willing within every ethnic and cultural group to receive God’s redemptive offer. God’s desire to quickly and efficiently bring redemption to a huge population explains why Earth’s recent history looks the way it does.
Endnotes
- At its birth, the Sun accumulated mass and brightened quickly—but during its youth it lost 20 percent or more of its mass, enough to cause more than a 100 percent loss of its luminosity. As it aged, the Sun’s nuclear furnace converted more and more hydrogen into helium, causing its luminosity to increase. The Sun continues brightening to this day and will one day generate enough heat energy to make Earth uninhabitable.
- About 40 million years ago, Antarctica separated from Australia and drifted south. About 23 million years ago, a wide strait began to form between Antarctica and the southern tip of South America, resulting in an open ocean surrounding Antarctica. The Antarctic circumpolar current and the settling of Antarctica upon the South Pole led to a thick ice sheet replacing the forests that once covered the continent.
- Stella C. Woodard et al., “Antarctic Role in Northern Hemisphere Glaciation,” Science 346 (November 2014): 847–51.
- Ibid.
- Bernhard Steinberger et al., “The Key Role of Global Solid-Earth Processes in Preconditioning Greenland’s Glaciation since the Pliocene,” Terra Nova (forthcoming; published online November 18, 2014): doi:10.1111/ter.12133.
- Ibid., first page.
- Prakash Kumar et al., “The Rapid Drift of the Indian Tectonic Plate,” Nature 449 (October 2007): 894–97.
- An Zhisheng et al., “Evolution of the Asian Monsoons and Phased Uplift of the Himalaya-Tibetan Plateau since Late Miocene Times,” Nature 411 (May 2001): 62–66; Warren L. Prell and John E. Kutzbach, “Sensitivity of the Indian Monsoon to Forcing Parameters and Implications for Its Evolution,” Nature 360 (December 1992): 647–52.
- Matthias Kuhle, “A Relief-Specific Model of the Ice Age on the Basis of Uplift-Controlled Glacier Areas in Tibet and the Corresponding Albedo Increase as well as Their Positive Climatological Feedback by Means of the Global Radiation Geometry,” Climate Research 20 (February 2002): 1–7.
- Zhisheng et al., “Evolution of Asian Monsoons,” 62–66.
- Nat Rutter and Z. Ding, “Paleoclimates and Monsoon Variations Interpreted from Micromorphogenic Features of the Baoji Paleosols, China,” Quaternary Science Reviews 12 (October 1993): 853–62.
- Shih Ya-feng et al., “Distribution, Features, and Variations of Glaciers in China,” in World Glacier Inventory, proceedings of the Riederalp Workshop, September 1978, IAHS-AISH publication no. 126 (1980): 111–16; R. V. Cruz et al., “2007: Asia,” in Climate Change 2007: Impacts, Adaptation, and Vulnerability, Contribution of Working Group II to the Fourth Assessment Report of the Intergovernmental Panel on Climate Change, ed. M. L. Parry et al. (Cambridge, UK: Cambridge University Press, 2007), 10.6.2; J. Graham Cogley, “Present and Future States of Himalaya and Karakoram Glaciers,” Annals of Glaciology 52 (December 2011): 69–73.
- Kuhle, “Relief-Specific Model,” 1–7; Zhisheng et al., “Evolution of Asian Monsoons,” 62–66; Lewis A. Owen and Jason M. Dortch, “Nature and Timing of Quaternary Glaciation in the Himalayan-Tibetan Orogen,” Quaternary Science Reviews 88 (March 2014): 14–54.
- Matthias Kuhle, “Reconstruction of the 2.4 Million km2 Late Pleistocene Ice Sheet on the Tibetan Plateau and Its Impact on the Global Climate,” Quaternary International 45–46 (1998): 71–108.
- Leopoldo D. Pena and Steven L. Goldstein, “Thermohaline Circulation Crisis and Impacts during the Mid-Pleistocene Transition,” Science 345 (July 2014): 318–22.
- Joseph J. Morley and Beth A. Dworetzky, “Evolving Pliocene-Pleistocene Climate: A North Pacific Perspective,” Quaternary Science Reviews 10, nos. 2–3 (1991): 225–37; Kuhle, “Relief-Specific Model,” 1–7; Matthias Kuhle, “The Tibetan Ice Sheet, Its Impact on the Palaeomonsoon and Relation to the Earth’s Orbital Variations,” Polarforschung 71, nos. 1–2 (2001): 1–13.
- Pena and Goldstein, “Thermohaline Circulation Crisis,” 318–22.
- Guocan Wang et al., “On the Geodynamic Mechanism of Episodic Uplift of the Tibetan Plateau during the Cenozoic Era,” Acta Geologica Sinica, English ed., 88 (April 2014): 699–716; Xiaomin Fang et al., “Oligocene Slow and Miocene-Quaternary Rapid Deformation and Uplift of the Yumu Shan and North Qilian Shan: Evidence from High-Resolution Magnetostratigraphy and Techonosedimentology,” in Magnetic Methods and the Timing of Geological Processes, eds. L. Jovane et al., Geological Society of London, Special Publications 373 (2013): 149–71.
- Kuhle, “Tibetan Ice Sheet,” 1–13; Kuhle, “Relief-Specific Model,” 1–7.
- Zaijun Li et al., “Chronology and Paleoenvironmental Records of a Drill Core in the Central Tengger Desert of China,” Quaternary Science Reviews 85 (February 1, 2014): 85–98; Zhengguo Shi et al., “Simulated Variations of Eolian Dust from Inner Asian Deserts at the Mid-Pliocene, Last Glacial Maximum, and Present Day: Contributions from the Regional Tectonic Uplift and Global Climate Change,” Climate Dynamics 37 (December 2011): 2289–301.
- Fang et al., “Oligocene Slow,” 149–71.
- Owen and Dortch, “Nature and Timing,” 14–54; Kuhle, “Reconstruction of the 2.4 Million,” 71–108; Kuhle, “Relief-Specific Model,” 1–7.
- Fang et al., “Oligocene Slow,” 149–71.
- A. S. Dyke et al., “The Laurentide and Innuitian Ice Sheets during the Last Glacial Maximum,” Quaternary Science Reviews 21 (January 2002): 9–31; Kuhle, “Tibetan Ice Sheet,” 1–13; Kuhle, “Relief-Specific Model,” 1–7.
- Shaun A. Marcott et al., “Centennial-Scale Changes in the Global Carbon Cycle during the Last Deglaciation,” Nature 514 (October 2014): 616–19; François Baudin, Nathalie Combourieu-Nebout, and Rainer Zahn, “Signatures of Rapid Climate Changes in Organic Matter Records in the Western Mediterranean Sea during the Last Glacial Period,” Bulletin de la Societe Geologique de France 178 (January 2007): 3–13; Thomas Blunier and Edward J. Brook, “Timing of Millennial-Scale Climate Change in Antarctica and Greenland during the Last Glacial Period,” Science 291 (January 2001): 109–12; Gerard C. Bond and Rusty Lotti, “Iceberg Discharges into the North Atlantic on Millennial Time Scales during the Last Glaciation,” Science 267 (February 1995): 1005–10.
- Peter J. Richardson, Robert Boyd, and Robert L. Bettinger, “Was Agriculture Impossible during the Pleistocene But Mandatory during the Holocene? A Climate Change Hypothesis,” American Antiquity 66 (July 2001): 387–411.